SynBio Series: An Investor's Guide to Navigating the Science of the Synthetic Biology Market
Unveiling the Fundamentals Concepts of Synthetic Biology
Dear Readers,
Welcome to Science Xplained - the newsletter that Empowers Investors to Navigate the World of Biotech Stocks with Scientific Precision! If you are new to SciX and want to get a view of what to expect in the articles, please click here.
In our previous articles, SciX took you on thrilling journeys through two emerging biotech trends: CAR Cell Therapy and Cultivated Meat. If you missed them, fear not! Our archived articles have got you covered.
But now, we're switching gears once again, diving headfirst into the exhilarating realms of the Synthetic Biology (SynBio) Market. Brace yourself as we kickstart the SynBio Series: An Investor's Guide, where we'll navigate the depths of SynBio's scientific landscape, starting with the Fundamental Concepts.
Stay tuned for subsequent articles that will delve into the major players shaping the SynBio landscape. We'll explore their research and development pipeline, uncover applications and market potential, unravel the regulatory landscape, conduct competitive analyses, assess team expertise, dissect market dynamics and trends, highlight upcoming events and conferences, and provide exclusive expert insights.
In a nutshell, the SynBio Series is your passport to gaining a deeper understanding of the captivating science behind the Synthetic Biology Market.
SynBio Series: Fundamental Concepts
This introductory article will explore the following:
Basic principles of SynBio, including its definition and
Delve into a timeline highlighting key milestones and events that shaped the field
You will discover why SynBio holds significant potential for investors and how it has gained widespread recognition and acceptance, particularly with the boost from the COVID-19 pandemic.
We will delve into the science behind various applications used in the production of synthetic life, providing a clear understanding of how SynBio works.
While SynBio offers immense opportunities, it is also a disruptive and rapidly evolving field. Therefore, we will discuss the areas where investors should exercise caution. We will explore the potential risks and challenges associated with SynBio, emphasizing the importance of diversification and adopting a long-term perspective to mitigate these risks.
1.1 What is Synthetic Biology ?
SynBio, short for Synthetic Biology, is an interdisciplinary field that combines biology, engineering, and computer science to design and construct new biological systems or modify existing ones. It involves applying engineering principles to biology, enabling scientists to create synthetic genetic circuits, pathways, and organisms with desired functions.
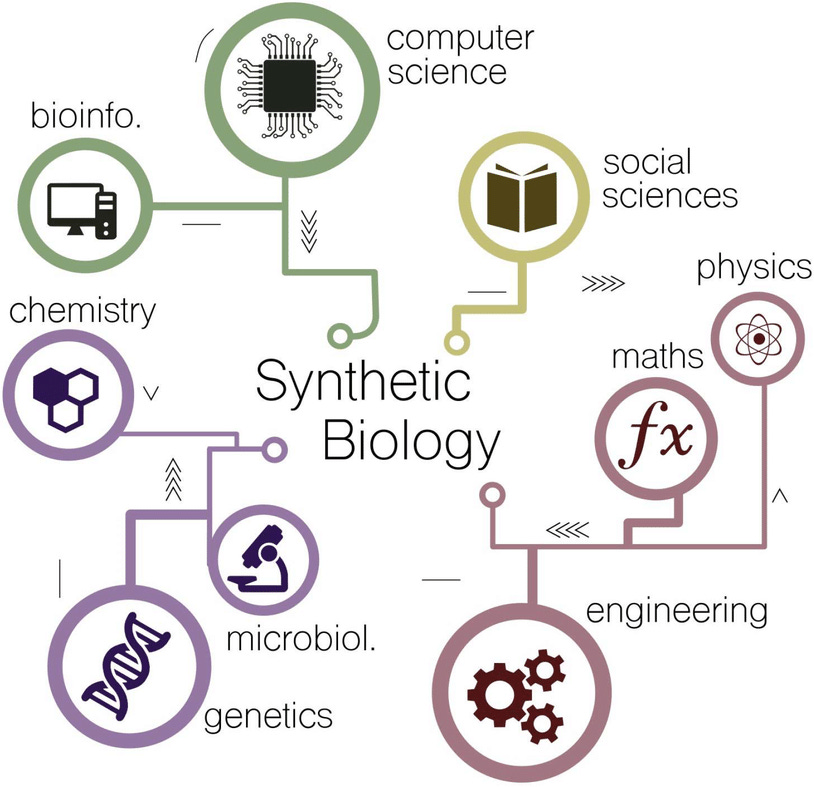
Microorganisms have long been our allies in various aspects of daily life. Traditionally, we have used microorganisms in bioprocessing to produce a range of products. For example, yeast has been employed for centuries to convert sugars into alcohol during fermentation, resulting in beverages like beer and wine. Similarly, certain strains of bacteria have been utilized to leaven bread, leading to fluffy loaves enjoyed worldwide. SynBio takes this concept further by leveraging advancements in DNA synthesis and genetic engineering. Instead of relying solely on the existing capabilities of microorganisms, scientists can now design and introduce new genetic codes into these organisms. These codes serve as blueprints that instruct the microorganisms to produce specific molecules or perform desired functions.
The potential applications of SynBio are vast. For instance, microorganisms can be programmed to produce biofuels as an alternative to fossil fuels, offering a more sustainable energy source. They can also be engineered to manufacture valuable chemicals, pharmaceuticals, and enzymes with improved properties and higher yields. By manipulating the genetic codes of microorganisms, SynBio opens up a world of possibilities for creating novel and beneficial products.
Through a combination of DNA synthesis, gene editing, metabolic engineering, and the design of genetic circuits, SynBio pioneers are working towards a future where microorganisms become versatile 'cell factories'. These engineered microorganisms can be designed to efficiently produce a wide range of substances, transforming industries and contributing to a more sustainable and technologically advanced society.
1.2 General timeline in the creation of SynBio
The field of synthetic biology has emerged and developed over several decades.
1970s-1980s: The advent of genetic engineering techniques and the discovery of recombinant DNA technology laid the foundation for manipulating and engineering genes.
In 1990s the field of genomics expanded with the completion of the Human Genome Project, which sequenced the entire human genome, providing a vast amount of genetic information for further study and manipulation.
In 2000, the term "synthetic biology" was coined by Dr. Stéphane Leduc, a French biologist.
In 2003, the first synthetic bacterial genome was created by Dr. Craig Venter's team at the J. Craig Venter Institute.
The field gained momentum with the development of standardized biological parts, known as BioBricks, by Dr. Tom Knight and colleagues at MIT.
In 2008, the International Genetically Engineered Machine (iGEM) competition was established, fostering collaboration and innovation in synthetic biology among students worldwide.
2010s: The field witnessed advancements in DNA synthesis technologies, making it easier and more affordable to create custom DNA sequences.
2012: The development of CRISPR-Cas9 gene editing technology revolutionized genetic manipulation and allowed for precise editing of DNA sequences. The scientists involved in this groundbreaking work were Jennifer Doudna and Emmanuelle Charpentier.
2020s: Synthetic biology continues to advance, with a focus on developing sustainable solutions, such as biofuels, bioplastics, and bioremediation.
2019-2022: The COVID-19 pandemic highlighted the rapid response capabilities of synthetic biology in vaccine development, including mRNA-based vaccines.
SynBio is a field that has been shaped by the contributions of numerous scientists, researchers, and entrepreneurs over the years. Few key figures that significantly contributed to the success of the field are Jay Keasling, Craig Venter, George Church, Tom Knight, Dre Endy, Christopher Voigt, Pam Silver, Ron Weiss, James Collins and many more. Their contributions have laid the foundation for the development of new applications, technologies, and approaches within synthetic biology.
Some nations have made significant contributions and are recognized for their strong presence in synthetic biology research. These countries include: US, UK, Germany, Singapore and China. Government of these countries have established initiatives like the Synthetic Biology Research and Development Programme and the Synthetic Biology Innovation Centre to drive research and industry collaborations. Also, various other countries, including Canada, Japan, Australia, and many European nations are contributing to the advancement of synthetic biology on a global scale.
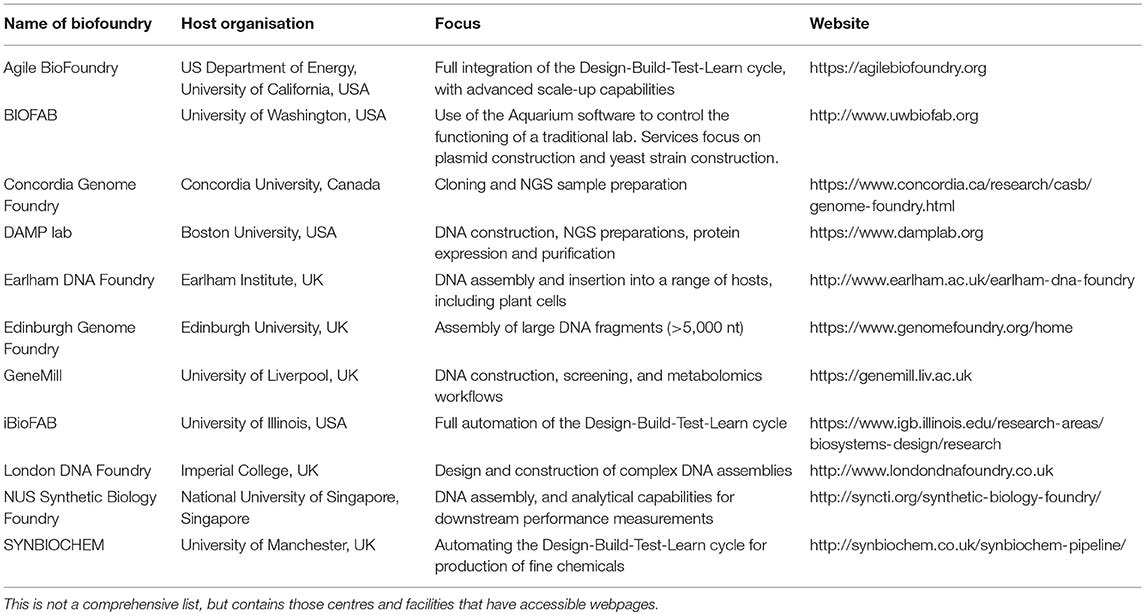
1.3 Why investors should take notice ?
The SynBio market is projected to reach USD 19.8 billion by 2025, exhibiting a remarkable compound annual growth rate (CAGR) of 23.9% compared to USD 6.8 billion in 2020. This remarkable progress is driven by the following key factors that make SynBio unique and forward-thinking:
1. The applications of SynBio are vast and diverse
SynBio represents a transformative and disruptive force in various industries, making it an essential sector for the future. It has been described as a disruptive technology at the heart of the so-called Bioeconomy, capable of revolutionizing various industries, including healthcare, agriculture, energy, materials, and environmental sustainability. In healthcare, it enables the development of new therapeutics, vaccines, and diagnostic tools that can revolutionize disease treatment and prevention. In agriculture, SynBio can enhance crop yield, improve nutritional content, and provide sustainable alternatives to conventional farming practices. Additionally, in energy, it has the potential to enable biofuel production from renewable sources, reducing dependence on fossil fuels. By addressing these challenges, SynBio aligns with global trends towards sustainability, health, and resource efficiency.
2. Addresses Market Demand of Sustainable and Eco-friendly solutions
The growing demand for sustainable and environmentally friendly solutions, coupled with the need for advancements in healthcare, agriculture, and energy, has created a market pull for SynBio innovations. SynBio has the potential to address complex challenges, improve productivity, and provide more sustainable alternatives, making it an integral part of the bioeconomy.
SynBio will enable the creation of biodegradable materials, advanced biofuels, bio-products, renewable chemicals, bio-based specialty chemicals, the development of eco-friendly manufacturing processes, and the exploration of alternative sources of food and energy.
3. Design-Build-Test-Learn Cycle: enhancing the potential for commercial success
The iterative and cyclical nature of the Design-Build-Test-Learn (DBTL) cycle in SynBio is an attractive aspect for investors. This iterative process involves designing genetic constructs, building them through DNA synthesis or genome editing, testing their functionality in living organisms, and learning from the results to improve the design. This cycle allows for rapid prototyping, fine-tuning, and optimization of biological systems, enabling faster innovation and reduced time to market for SynBio products. The DBTL approach provides investors with confidence that companies can iterate and improve their technologies, enhancing the potential for commercial success.
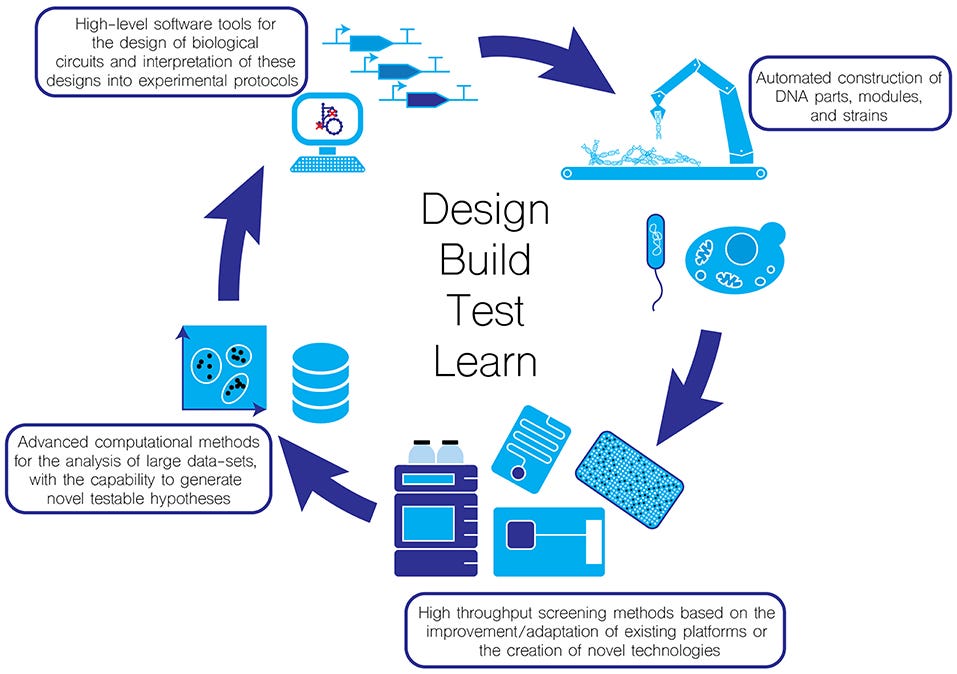
4. Cell-free systems (CFS) are expanding SynBio’s applications.
Cell-free systems have emerged as a transformative approach in synthetic biology, offering distinct advantages over traditional cell-based systems. While cell-based systems have made significant progress, they come with inherent limitations. In contrast, cell-free systems, often referred to as programmable liquids, have revolutionized the field by simplifying and expanding the possibilities of designing and manipulating biological systems.
Cell-free systems have found numerous applications in synthetic biology. They have facilitated rapid protein synthesis. Moreover, cell-free systems are instrumental in pathway engineering and biosynthesis. Researchers can reconstitute specific metabolic pathways in vitro, enabling the production of complex molecules like pharmaceuticals, biofuels, and specialty chemicals. Another significant advantage of cell-free systems lies in their utility for prototyping genetic circuits and synthetic gene networks. In vitro construction and testing of genetic circuits allow for rapid iteration and optimization, accelerating the development of genetic devices and synthetic biology applications.
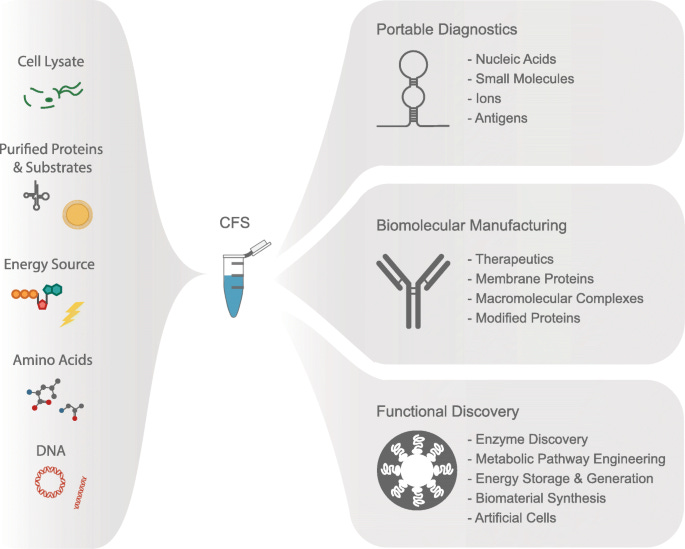
With their simplified and programmable nature, cell-free systems offer new avenues for rational design and manipulation of biological systems.
5. Intellectual Property and Patent Landscape
SynBio presents a dynamic intellectual property landscape, offering attractive opportunities for investors. Patents covering foundational technologies, unique genetic constructs, and novel applications form valuable assets for SynBio companies. Intellectual property protection can provide market exclusivity, create barriers to entry for competitors, and generate licensing or royalty revenue streams. Investors are keen to evaluate the strength and breadth of a company's intellectual property portfolio, as it can significantly impact the company's competitive advantage and market potential.
Considering these factors, SynBio presents an attractive investment opportunity. Its disruptive capabilities, market potential, alignment with global challenges, sustainability focus, policy support, and increasing investor interest make it an essential sector for future investments. By strategically investing in SynBio companies, investors can participate in the advancements shaping our future, drive positive impact, and potentially achieve significant financial returns.
1.4 Factors that contributed to the accessibility of SynBio
1. Declining Costs of DNA Synthesis
SynBio has been propelled by significant technological advancements. One notable example is the dramatic reduction in the cost of nucleotide sequencing, which is a critical component of understanding and manipulating DNA. The advent of high-throughput sequencing technologies, such as Next-Generation Sequencing (NGS), has revolutionized genomic analysis and made it more accessible. The plummeting costs of sequencing have opened doors for broader adoption, enabling researchers and companies to generate vast amounts of genomic data at a fraction of the previous expenses. For example, in molecular biology, the cost of nucleotide sequencing has dropped from nearly $USD10,000 per megabase in 2001 to $US0.012 in July 2017.
2. Technological Advancements
Rapid advancements in DNA synthesis, gene editing techniques, automation and robotic systems have significantly reduced the cost and time required for genetic engineering. High-throughput DNA synthesis platforms, such as microarray-based synthesis and oligonucleotide pool-based synthesis, enable the rapid assembly of DNA fragments, genes, and even entire genomes. CRISPR-Cas9 have made genome editing faster, more efficient, and highly targeted. Automated liquid handling systems, robotic platforms, and high-throughput screening methods enable the manipulation, culturing, and analysis of large numbers of biological samples. These technologies streamline the experimental process, increase reproducibility, and accelerate the development of synthetic organisms and engineered products.
3. Computational Modelling, Data Analytics and Machine Learning
The integration of computational modelling and machine learning has revolutionized the field of SynBio. Sophisticated algorithms, simulation software, and predictive models allow researchers to design and optimize biological systems before they are physically constructed. Additionally, machine learning algorithms can identify patterns, correlations, and predictive models from large-scale genomic, transcriptomic, proteomic, and metabolomic datasets. This approach saves time and resources by guiding experimental design and improving the success rate of genetic engineering projects.
4. Cloud Computing and e-Science
Cloud platforms provide researchers and companies with scalable computational resources to analyze massive datasets, simulate complex biological systems, and perform computationally intensive tasks. E-science emphasizes the management, analysis, and interpretation of large and complex datasets. It involves the use of data mining, machine learning, and other computational techniques to extract meaningful insights from vast amounts of data. This approach allows researchers to uncover patterns, correlations, and trends that may not be readily apparent using traditional methods.
5. Open-Source Collaboration
The culture of open-source collaboration and information sharing within the SynBio community has played a vital role in fostering innovation and driving accessibility. Initiatives such as the BioBricks Foundation and the sharing of standardized biological parts (BioBricks) have accelerated progress by enabling researchers to build upon existing knowledge and tools.
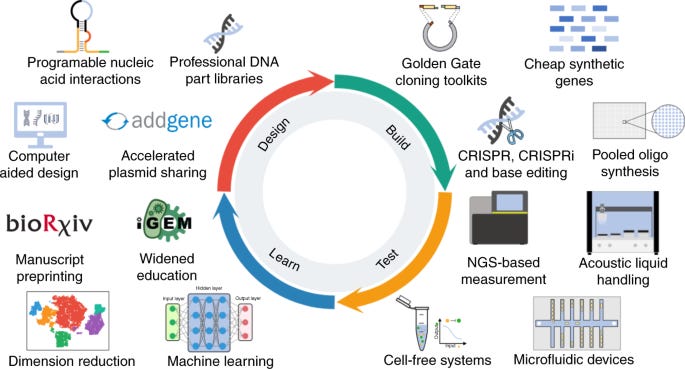
1.5 COVID-19 pandemic propelled SynBio Revolution
SynBio, barely 20 year old discipline played multiple roles at the forefront of the global pandemic response.
Vaccine Development: Messenger RNA (mRNA) vaccines, such as the Pfizer-BioNTech and Moderna vaccines, utilize SynBio techniques to deliver the genetic instructions for producing the spike protein of the SARS-CoV-2 virus. This innovative approach allowed for the accelerated development and production of effective vaccines.
Antiviral Therapies: Researchers have used computational modeling and drug discovery techniques to identify potential antiviral drugs and repurpose existing ones for COVID-19 treatment.
Antibody Engineering: SynBio has facilitated the engineering of antibodies for COVID-19 treatment and prevention. Researchers have utilized synthetic biology techniques to produce monoclonal antibodies that specifically target the SARS-CoV-2 virus. These engineered antibodies have shown promise in neutralizing the virus and reducing the severity of COVID-19 symptoms.
Biosensors and Surveillance: SynBio has contributed to the development of biosensors and surveillance systems for monitoring COVID-19 spread. Researchers have utilized genetic engineering and synthetic biology methods to create biosensors that detect the presence of the SARS-CoV-2 virus or its antigens in environmental samples. These biosensors have been employed in wastewater monitoring and environmental surveillance to track the prevalence and spread of the virus in communities.
Bioproduction and Supply Chain Optimization: SynBio approaches have been utilized to optimize bioproduction processes and address supply chain challenges related to COVID-19. Researchers have employed genetic engineering techniques to enhance the production of critical therapeutic proteins, enzymes, and reagents necessary for COVID-19 testing and treatment. SynBio has also played a role in developing sustainable and scalable biomanufacturing processes to meet the increased demand for medical supplies during the pandemic.
1.6 Standard techniques used in SynBio Industry
Let’s now explore a hypothetical SynBio application that incorporates several major standard techniques used in the field.
Imagine a SynBio company working on developing a sustainable and eco-friendly method to produce a valuable compound called "BioPlast." BioPlast is a biodegradable plastic alternative that can help reduce the environmental impact of traditional plastics. To achieve this, the company employs various SynBio techniques: machine learning algorithms, DNA Synthesis and Gene Assembly, Genome Engineering and Gene Editing, Metabolic Engineering, Biosensors and Genetic Circuits, Phenotypic Assays, Analytical Chemistry Techniques, Transcriptomics and Proteomics, Metabolomics, Next-Generation Sequencing (NGS).
Taking this hypothetical example of BioPlast, let’s dig deeper to get familiar with the science of the above mentioned techniques. For clarity we can breakdown the whole production process into different phase:
In silica Design and Gene Selection: The initial phase involves using computational tools and in silico techniques to identify and select specific gene codes relevant to BioPlast production. The company feeds large amounts of genomic and biochemical data into machine learning models, training them to recognize patterns and identify genetic sequences that are likely to enhance BioPlast production. By analyzing vast datasets of genetic information and metabolic pathways, the machine learning algorithms can predict which combinations of genetic modifications are most likely to yield improved enzyme activity and pathway efficiency. These codes may involve combinations of genetic elements from different organisms or entirely synthetic sequences that have been optimized for BioPlast production. Traditional methods of genetic engineering often involve a trial-and-error approach, but with the aid of machine learning algorithms, the process becomes more efficient and targeted.
This step allows for targeted selection and customization of genes for the subsequent engineering steps.
Microorganism Selection: Once the specific gene codes are identified, the next step is to choose the appropriate microorganism as the host for the genetic modifications. This selection is based on various factors such as the organism's ability to tolerate genetic manipulation, its metabolic capabilities, and its compatibility with the desired production conditions. Microorganisms like bacteria or yeast are commonly used due to their well-characterized genetic systems and ease of manipulation.
Synthesis and Genetic Engineering: The company designs and synthesizes DNA sequences encoding enzymes involved in the biosynthesis of BioPlast. These enzymes are not typically found in naturally occurring organisms but are derived from other sources or engineered for optimal performance. The company uses gene editing techniques, such as CRISPR-Cas9, to precisely modify the genetic code of microorganisms, such as bacteria or yeast, to enhance their ability to produce the desired compounds involved in BioPlast synthesis. This may involve introducing specific genetic modifications to increase enzyme activity or alter metabolic pathways.
Metabolic Engineering: The company applies metabolic engineering principles to optimize the metabolic pathways of the microorganisms. This involves introducing additional enzymes or modifying existing ones to improve the production efficiency and yield of the key building blocks required for BioPlast synthesis.
Biosensors and Genetic Circuits: The company incorporates biosensors into the engineered microorganisms to monitor the production process and ensure optimal conditions for BioPlast synthesis. These biosensors detect specific molecules or environmental signals, enabling real-time feedback and control of the production process. Genetic circuits may also be implemented to fine-tune gene expression and regulate the metabolic pathways for efficient BioPlast production.
Small-Scale or "pilot-scale" Production Phase: After genetic engineering, the modified microorganisms are cultivated and grown in small-scale laboratory setups. These cultures serve as a testing ground to assess the performance of the engineered strains. It involves Phenotypic Assays, Analytical Chemistry Techniques, Transcriptomics and Proteomics, Metabolomics, and NGS techniques for optimizing growth conditions, evaluating production yields, and ensuring the stability and functionality of the modified microorganisms. Small-scale production allows for fine-tuning and troubleshooting before scaling up to larger volumes.
Phenotypic Assays: Phenotypic assays are used to evaluate the observable characteristics or behaviors of the engineered microorganisms. This may involve assessing the production yield of the desired product, such as BioPlast, by quantifying its concentration or measuring its physical properties. Phenotypic assays can also include growth rate measurements, substrate utilization assays, or response to specific environmental conditions.
Analytical Chemistry Techniques: Analytical chemistry techniques are used to analyze and quantify the metabolites, enzymes, and other molecular components involved in the production process. These techniques may include high-performance liquid chromatography (HPLC), gas chromatography-mass spectrometry (GC-MS), nuclear magnetic resonance (NMR), or spectrophotometry. They help in understanding the metabolic profile of the microorganisms and determining the efficiency of the production pathway.
Transcriptomics and Proteomics: Transcriptomics involves studying the RNA transcripts present in the engineered microorganisms, providing insights into gene expression levels and identifying any changes induced by the genetic modifications. Proteomics, on the other hand, focuses on analyzing the proteins produced by the microorganisms. Transcriptomic and proteomic analyses can reveal how the engineered microorganisms are behaving at the molecular level and help optimize their performance.
Metabolomics: Metabolomics involves profiling and analyzing the small molecules or metabolites present within the engineered microorganisms. By comparing metabolite profiles of different strains or conditions, metabolomics can provide a comprehensive understanding of the metabolic pathways and identify any bottlenecks or areas for improvement.
Next-Generation Sequencing (NGS): NGS techniques, such as whole-genome sequencing or targeted sequencing, can be employed to verify the genetic modifications made to the microorganisms. This helps ensure the stability and integrity of the engineered strains and provides a comprehensive view of their genetic makeup.
Standard techniques used in SynBio | Taken from Research Article
1.7 Risks associated with SynBio
While SynBio presents numerous opportunities, it is important for investors to be aware of the potential drawbacks and risks associated with the field. Here are some cons of SynBio that investors should consider:
Technical Challenges: Achieving desired outcomes may require extensive research, development, and iterative improvements, which can result in delays and increased costs.
Regulatory Hurdles: The regulatory landscape for SynBio is still evolving, and navigating the complex regulatory frameworks can be challenging. The introduction of novel synthetic organisms and genetically modified organisms (GMOs) may require regulatory approval, which can be time-consuming and costly. Changes in regulations or unforeseen regulatory barriers could impact the development and commercialization of SynBio products.
Ethical and Societal Concerns: The field of SynBio raises ethical considerations regarding the creation and manipulation of living organisms. Controversial topics such as synthetic life forms, gene editing, and potential unintended consequences of genetic modifications can raise public concerns and regulatory scrutiny. Investor perception and public acceptance of SynBio applications could influence market dynamics and adoption rates.
Intellectual Property Issues: Intellectual property protection is crucial in the biotech industry, including SynBio. However, patenting complex biological systems and synthetic organisms can be challenging due to the inherent nature of living systems. Patent disputes, licensing agreements, and the potential for intellectual property challenges can impact the competitive landscape and profitability of SynBio companies.
Market Competition and Uncertainty: SynBio is a rapidly growing field, and market competition is increasing. The emergence of new players, disruptive technologies, and evolving market dynamics can create uncertainties. Investing in SynBio requires careful assessment of competitive advantages, market positioning, and the ability of companies to adapt to changing market conditions.
Environmental and Biosafety Risks: Introducing synthetic organisms into the environment can pose potential environmental and biosafety risks. Although strict biosafety protocols are in place, the accidental release or unintended consequences of genetically modified organisms could have ecological implications. Investors should consider the potential environmental and reputational risks associated with SynBio companies.
It is important for investors to conduct thorough due diligence, understand the technical and regulatory challenges, and assess the risk-reward profile of SynBio investments. Diversification and a long-term perspective can help mitigate risks associated with this rapidly evolving field.
If you're enjoying our content and want to dive deeper into the science of Synthetic Biology, consider upgrading to our premium subscription. With the premium version, you'll have access to subsequent articles in SynBio Series: An Investor's Guide to navigate the science of SynBio Market.
To give you a taste of what's in store for the SynBio series, we will delve into the world of major players shaping the SynBio landscape. Join us as we explore their research and development pipeline, uncover applications and market potential, unravel the regulatory landscape, conduct competitive analyses, assess team expertise, dissect market dynamics and trends, highlight upcoming events and conferences, and provide exclusive expert insights.
Which synthetic biology company are you particularly interested in for an in-depth scientific analysis? Let us know, and we'll ensure to provide you with comprehensive insights and analysis tailored to your specific interest.